1. INTRODUCTION
With governments and
industry wanting to develop clean hydrogen,
there is an opportunity to initiate
exploration projects for hydrogen (and also
helium) from natural sources in regions like
Témiscamingue (Quebec). The search for
hydrogen (H2) and helium (He) sources is
relatively new, which explains the lack of
geoscientific information available on
government web sites such as SIGEOM (MRN).
However, large corporations, such as Total
(France), finance vast exploration projects
(e.g. in the Pyrenees) for the search for
natural hydrogen (Dugamin et al., 2019).
The prospecting methods (geochemistry,
geophysics) used for hydrogen exploration
are similar to those used in geothermal
energy and for natural gas exploration (Tian
et al., 2022). For companies like Quebec
Innovative Materials Corporation (QIMC),
there would be real opportunities in this
area, because during exploration work for
natural hydrogen (or native hydrogen), it is
also likely to find helium and also
geothermal heat sources. Economic
projections show that global hydrogen
consumption is expected to increase in the
coming years (Fig. 1). This is an
opportunity for QIMC to position itself
relatively early in the process of
exploration and possibly production of
hydrogen and/or helium in Quebec.
The principle of He-H2
exploration is, as for natural gas, to find
reservoirs rich in hydrogen or helium and to
exploit them commercially from the surface
(wells) (Milkov, 2022). Hydrogen and
helium being gases often associated in
nature, it is also possible to exploit
helium which is a rare industrial gas.
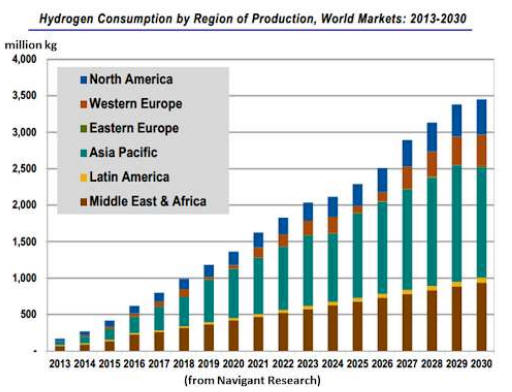
Figure 1. World
evolution of hydrogen consumption for the
period 2013-2030. Source:
http://newenergynews.blogspot.com/2013/11/world-still-building-hydrogen-hiway.html.
2. LOCATION OF
EXPLORATION WORKS FOR HYDROGEN AND HELIUM
Given the lack of
geoscientific data on hydrogen and helium
from natural sources in Quebec and also for
most of the Canadian territory, QIMC
proceeded to a judicious selection of
exploration permits based on current global
knowledge on the geology of hydrogen and
helium showings in the world. First, regions
combining Precambrian bedrock rich in
potassic rocks (radiogenic) and Paleozoic
sedimentary rocks were prioritized. This
type of geological environment is known to
be able to form H2 and He. In addition to
these lithological features, QIMC searched
for regions affected by rift and graben
tectonic systems. The latter are
particularly well developed in eastern
Quebec. The Ottawa-Bellechere and Lake
Témiscamingue grabens (Fig. 2 and 3)
are tectonically active structures as
evidenced by the presence of continental
intraplate seismicity well documented by the
Geological Survey of Canada.
Extensional geological
structures, such as the Temiscamingue graben
system (Fig. 2 and 3), are known to
contain normal faults that can focus gas
circulation to the surface. The presence of
a cover of Proterozoic (Cobalt Group) and
Paleozoic (New Liskard) Ordovician
sedimentary rocks is important in order to
provide potential storage sites (reservoirs)
and impermeable covers limiting the
dispersion of gases towards the surface. The
presence of serpentinized ultramafic rocks
(altered komatiites and dunites) and Archean
iron formations (Baby Group) beneath the
sequence of sedimentary rocks is a potential
favourable source for hydrogen production.
The presence of a thick sequence of arkosic
rocks in the Cobalt Group and of Precambrian
potassic granitoids in the Lake
Témiscamingue area are potential sources of
helium production (sources rich in U and Th).
Figure 4 shows
the spatial distribution of QIMC exploration
permits in the municipality of St-Bruno-de-Guigues
(MRC du Témiscamingue).
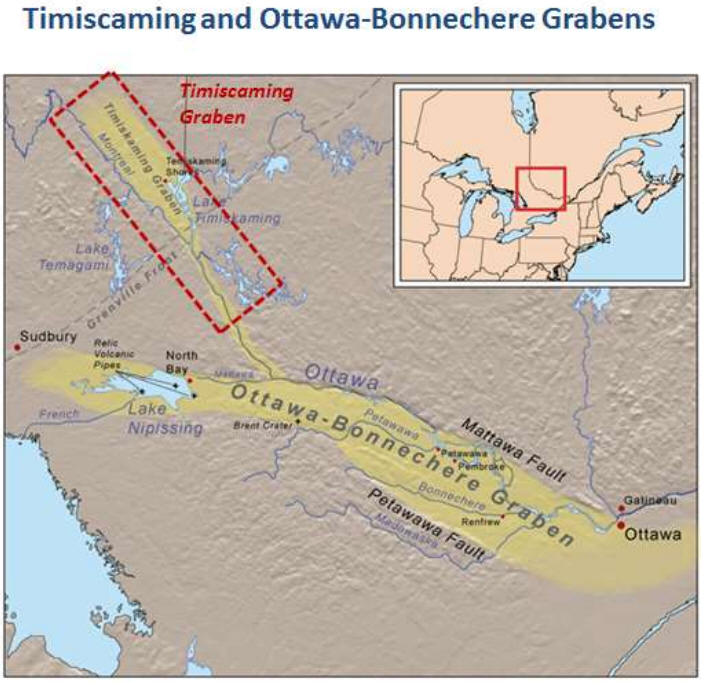
Figure 2.
Tectonic zones of the Ottawa-Bonnechere and
Timmiskaming (or Témiscamingue in French)
grabens (Quebec and Ontario). The QIMC
exploration area is located in the
Timmiskaming graben zone.
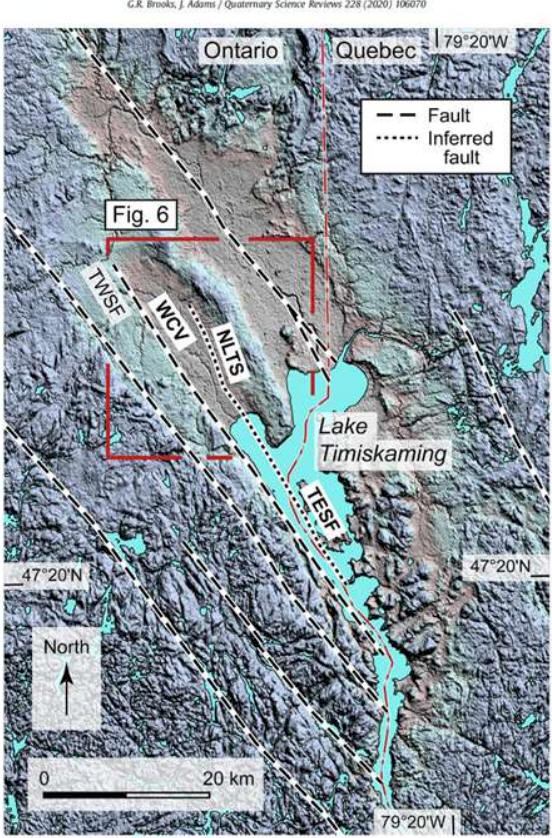
Figure 3. Map of
the Timiskaming graben located on the border
of Quebec (east) and Ontario (west). Some
faults on the Ontario side of Lake
Timiskaming are shown and correspond to
topographic escarpments.
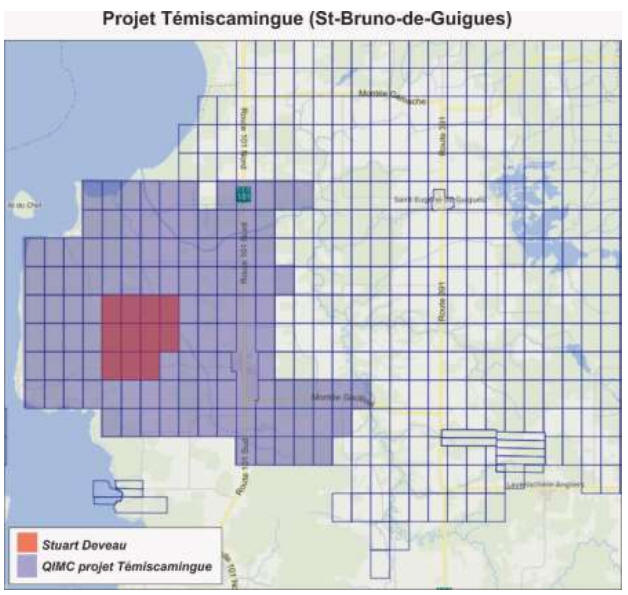
Figure 4. Map of
QMIC exploration permits located in the area
of the municipality of Saint-Bruno-de-Guigues
(MRC du Témiscamingue). Modified from the
MRN website (SIGEOM). Note that the Stuart
Deveau claim area was re-staked by QIMC on
May 6, 2023.
3. GEOLOGICAL CONTEXTS
FAVOURABLE TO THE PRESENCE OF HYDROGEN AND
HELIUM
3.1 GEOLOGICAL AND
GEOCHEMICAL PROCESSES INVOLVED IN THE
FORMATION OF HELIUM
Helium-rich fields can
be subdivided into three categories. Those
rich in methane, associated with natural gas
deposits, those associated with nitrogen
(N2) and those associated with CO2 (Liu
et al., 2023). The close association of
He-CO2 and He-N2 explains the importance of
measuring CO2 and N2 during prospecting work
for helium. Instrumentally, it is very easy
to measure CO2 and N2 concentrations
directly in the field, while helium
measurement is more complex and requires, in
many cases, the use of a specific mass
spectrometer. Unlike methane, helium-rich
reservoirs can be considered economical from
low concentrations such as 0.3% (3000 ppm).
Deposits associated with N2 have recently
been discovered in the Colorado plateau
(USA) and in the Tancheng- Lujiang fault
zone in China. Those rich in CO2 has been
discovered, among other places, in the
Colorado Plateau and in the Western
Cordillera (Halford et al., 2022). It
is noteworthy that the helium deposits in
production are mainly present in Paleozoic
rocks. However, several deposits are also
present in Proterozoic rocks. In contrast,
there is no helium deposit in production in
Archean rocks. The global compilation by
Liu et al. (2023) shows that reservoirs
can be located at depths of 0 to 3 km with a
majority of reservoirs located at depths
between 1 and 2 km. Note that deposits
located between 0 and 1 km are almost as
common as those located between 2 and 3 km
deep. In the context of exploration in the
Témiscamingue area, the abundance of
Proterozoic sedimentary rocks (Cobalt Group)
and the presence of Ordovician limestone and
detrital rocks (quartzite and sandstone)
near Lake Timiskaming constitute relevant
exploration targets for helium exploration.
The local geological
context is a key factor in an exploration
strategy for H2 and He. In addition to
considerations of reservoirs (porous rocks)
and impermeable barriers (e.g. shales),
rocks likely to produce the desired gases
must be found in the region. For helium,
this gas can come in small proportion of
mantle sources ( 3He). The latter is often
observed in active volcanic terrain. This
primitive (or primordial) gas is added to
4He (distinctly dominant) which comes from
crustal sources via the alpha decay of the
natural occurring of radiogenic isotopes of
uranium (235U and 238U) and thorium (232Th)
(Fig. 5).

Figure 5. Alpha
decay of a uranium-238 nucleus and
production of radiogenic helium.
Unlike hydrogen, helium
is an inert gas and therefore non-reactive.
Its solubility in water is relatively low
and this gas migrates easily to the surface
or to reservoirs that can accumulate it over
long periods of time. Unlike hydrocarbons,
when present in the area, helium continues
to form due to the very long half-lives of
uranium and thorium isotopes. Rocks rich in
U and Th are mainly felsic and potassic in
nature, such as Precambrian felsic granites
and gneissic rocks. These rocks are
particularly abundant in the Canadian Shield
in Quebec and Ontario (e.g. Témiscamingue,
Lac St-Jean and Charlevoix). In addition,
certain sedimentary rocks such as arkose are
also recognized as potential sources of
helium production. These rocks are very
abundant in the succession of Proterozoic
Huronian sedimentary rocks of the Lake
Témiscamingue region.
In continental
environments, many of the largest helium
accumulations are observed in cratonic
terrains such as the Canadian Shield. These
vast Precambrian terrains are tectonically
stable environments over very long periods
of time. Late extensional environments such
as rifts and grabens (e.g., Timiskaming and
Saguenay graben) can facilitate gas
migration to shallow reservoirs or the
surface, via vast networks of faults (Fig.
6).

Figure 6.
Simplified geological section of the
geological structures and formations
associated with the Saguenay Graben.
3.2 GEOLOGICAL AND
GEOCHEMICAL PROCESSES INVOLVED IN THE
FORMATION OF HYDROGEN
The observation of
hydrogen leaks in the ground is a phenomenon
frequently observed on the surface of the
Earth. The phenomenon is observed in rocks
from different geological periods and in a
multitude of geological environments. The
work carried out on the edge of the Pyrenees
Mountains belt (France) is a good example
showing the presence of significant regional
hydrogen anomalies observed in the soils of
the Pau region (Fig. 7).
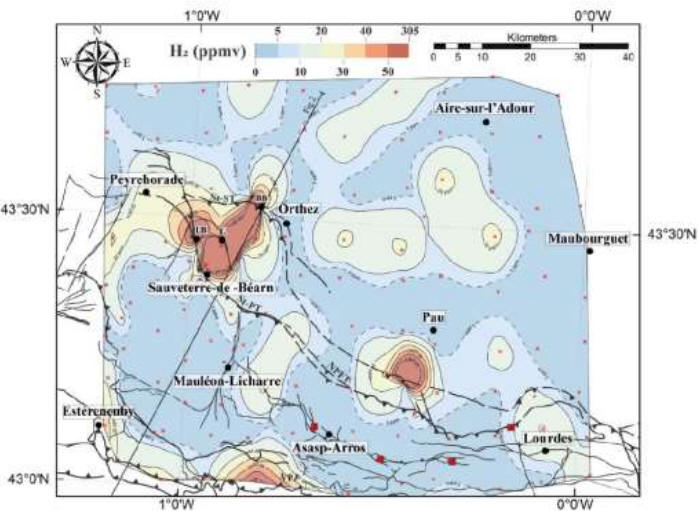
Figure 7. Map
showing the spatial distribution of hydrogen
anomalies measured in the soils of the Pau
region (Pyrenees, France). Note the spatial
association with regional faulting and
hydrogen concentrations. Map taken from
Lefeuvre et al. (2021).
Unlike helium, this
observation highlights that a multitude of
geochemical processes can form hydrogen. The
article by Milkov (2022) lists some
thirty physical and chemical mechanisms that
can lead to the production of natural
hydrogen. The main mechanisms that can lead
to the production of hydrogen can be grouped
into abiotic or biotic processes. The
abiotic processes include: 1) degassing of
primordial hydrogen from ultra-deep sources
in the Earth's mantle, 2) radiolysis of
water by radioactive elements (U, Th, K)
present in the Earth's crust and 3)
interactions between water and rock minerals
(mostly iron-rich minerals). Biotic
processes involve the degradation of organic
matter in rocks (source of hydrocarbons) as
thermal maturity increases (Mahlstedt et
al., 2022) and also bacterial processes
that can produce hydrogen (e.g., anoxic
peatlands).
In geologic materials,
oxidation of iron-rich rock minerals (Fe2+)
such as iron formations (magnetite-rich
facies) and ultramafic rocks (e.g.,
peridotites and ophiolites) can generate
hydrogen (Tian et al., 2022). This is
particularly well demonstrated in Turkey by
the Chimaera gas seepage, which emits
natural hydrogen and has fuelled “eternal”
flames for thousands of years (Etiope,
2022). Despite the fact that the
majority of hydrogen observed at the surface
comes mainly from chemical reactions
involving fluids and minerals, certain
accumulations of hydrogen come from the
radiolysis of water or hydrocarbons (ex.
CH4) by natural radioactive elements present
in certain geological formations. Potassic
granite, arenite (e.g., potassic sandstone
from the Cobalt Gp in Témiscamingue) and
evaporite (e.g., Iles-de-la-Madeleine) are
crustal rocks capable of producing hydrogen
by radiolysis.
4. METHODOLOGY
INRS conceptual
exploration approach is based on a
multidisciplinary study involving, among
other things, the geochemistry of gases
in the vadose zone of soil (soil not
saturated with water), the chemistry of
atmospheric gases measured at very low
elevations above the ground, geophysics
(gravity, audiomagnetotellurics),
structural geology and drone imagery
(Lidar, SFM, multispectral).
In order to maximize
the probability of discovering hydrogen and
helium leaks, it is necessary to locate
faults and other geological discontinuities.
In Quebec, these structures are most often
masked by Quaternary glacial deposits and
lake sediments. Being difficult to observe,
these structures are most often regionally
extrapolated or inferred from fragmented
geological data or from imprecise indirect
methods (e.g., Quebec Government
low-resolution magnetic map). On the other
hand, CO2, CH4, radon (and thoron), He, H2
are gases frequently used to highlight the
presence of faults masked by overburden (e.g.,
Toutain and Baubron, 1999). Similarly,
mobile gamma radiometric measurements (on
the ground) can be used to locate uranium
enrichment halos often testifying to the
presence of degassing faults. These halos
form by precipitation of uranium from
groundwater when the gas concentration
becomes high, favouring the formation of
reducing media.
Lidar (airborne laser)
is useful for locating local linear
topographic breaks indicating the presence
of recent (neotectonics) or old faults.
Finally, geophysical methods with high
vertical penetration such as
audiomagnetotellurics (AMT) can locate and
assess the importance of structures observed
on the surface (vertical penetration of
0-2000m). Typically, for gases like helium,
the greatest outgassing comes from
deep-rooted faults in the continental crust.
4.1 GEOCHEMISTRY AND
DYNAMICS OF SUBSURFACE GAS SEEPAGES
At the Earth's surface,
gases are transferred to the atmosphere via
diffusive (slow) processes and locally by
advective (fast) processes (Fig. 8).
For regional exploration, anomalous areas
are most often identified by preliminary
Soil-Gas surveys that are sensitive for
locating diffuse sources. Subsequently,
anomalous zones in H2 or He are subject to
high-resolution gas measurements to assess
the gas fluxes from advective sources. These
sources bring large volumes of gas to the
surface (degassing zone).
At the regional scale,
it is possible to measure gas concentrations
present in the vadose zone in order to
locate anomalous areas potentially
favourable to the presence of gases such as
helium and hydrogen. This is demonstrated,
among other things, by the study of helium
distribution on the volcanic island of
Santorini in Greece (Fig. 9).
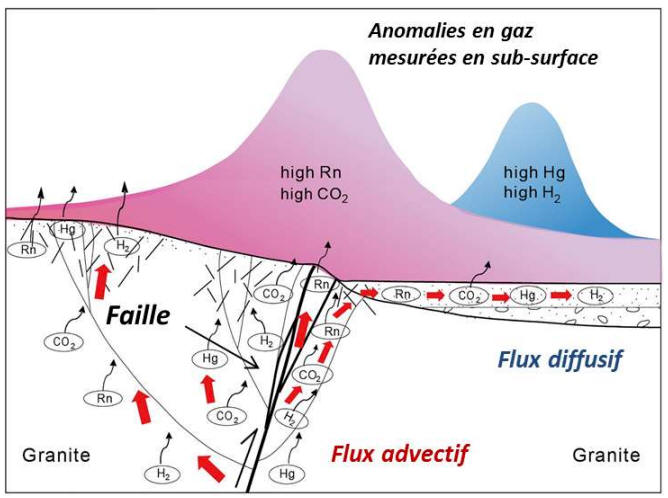
Figure 8.
Advective and diffusive fluxes of radon (Rn),
CO2, H2 and Hg in the vicinity of a fault
zone cutting granitic rocks.

Figure 9. Helium
and CO2 concentrations in soils from the
island of Santorini (Greece). Source:
Tarchini et al. (2019). The Soil-Gas
method is effective in locating areas of
interest (diffuse sources) for the
exploration of gases such as helium.
4.1.1. Significance of
regional faults
The Temiscamingue
graben area is severely affected by
seismicity and normal faulting related to
extensional processes still active today (Fig.
10). Such structures may be important in
allowing the transfer of gases from deep
sources to shallow environments. For H2 and
He exploration, these faults must be well
located in space (Lidar, AMT, gravity,
magnetometric surveys) and geophysical
surveys must be carried out in order to
verify the vertical extension (in depth) of
the faults. The deeper these structures are,
the greater the potential for gas transfer

Figure 10.
Schematic block diagram showing normal
faulting (collapse) associated with the
Témiskaming graben.
Due to high porosity
and permeability, fault zones promote gas
focusing and advection within a relatively
restricted area of fractured rocks (Fig.
8). The advection of gases, in such an
environment, results in a significant
transfer of gases towards the surface
(flux). To detect areas of potential gas
advection, it is necessary to measure gas
flows in the field (concentrations per unit
area / time) with devices specially designed
for this purpose. As mentioned in this
document, fundamental structures such as
faults (and shear zones) are more permeable
to gas than intact rock. In many cases, this
allows the formation of detectable gas
leaks. In the presence of a porous structure
in the rock (e.g. Témiscamingue lake
dolomitized Ordovician limestone) and an
impermeable cover above (e.g., shale,
basalt, marine or glaciolacustrine clays),
these gases can form potentially exploitable
accumulations (Fig. 11).
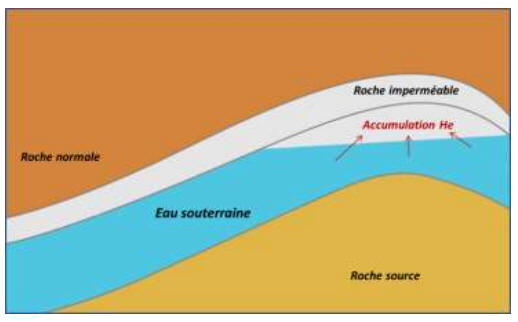
Figure 11.
Conceptual vertical section showing the
accumulation of helium in a porous
geological structure surmounted by the
presence of a barrier of impermeable rocks
(shale).
Sometimes, on the
surface, these gas leaks can form
sub-circular structures with little
vegetation (detectable by Lidar) but most of
the time these leaks are cryptic and require
soli-gas analysis in order to be able to
locate them in the field. The use of
multi-spectral cameras on drones is an often
effective indirect method for locating
degassing areas in forest environments. The
method detects stresses in tree vegetation
related to the depletion of oxygen in the
soil and changes in pH (acidity) and redox
potential related to the presence of gas
leaks. For example, the use of the
Agrowing multispectral lens interface
with the ADTI 61 Mpixel camera makes
it possible to cover large areas in wooded
areas.
4.1.2. Chemical
analysis of gases
The evaluation of the
gas transfer mode requires the use of
optimized methods to measure the diffusive
or advective fluxes. For H2 and He
exploration, two gas analysis methods should
be used. The first is a Soil-Gas prospecting
method which consists of analyzing gases
present in the soil and more precisely at a
depth of 1m (detection of diffusive
anomalies) and the other is a method for
measuring gases flux present immediately
above the soil-atmosphere interface
(detection of advective flows).
4.1.2.1 Gas analysis at
the soil-air interface and advective flux
measurements.
This method is based on
the measurement of gases present immediately
above the soil-atmosphere interface.
Measurement in contact with the ground makes
it possible to minimize the dilution by
atmospheric gases. The technology that could
be used in the second year of the project
for this analytical procedure is based on
laser optical cavity spectroscopy allowing
the simultaneous measurement of CO2,
CH4 (Li-Cor-7810). To
locate areas of gas advection in the field,
it is necessary to use ultra-sensitive
systems (ppb detection limits) capable of
measuring gas concentrations continuously
over variable periods of time. Helium and
hydrogen field analyzers are not designed
for such continuous operation. On the other
hand, carrier gases such as CO2
and CH4 should be measured in
the field because they are often associated
with hydrogen and helium seepages.
Since CO2,
CH4 and Hg are very
sensitive for locating geological fault it
could be possible to carry out a very large
number of measurements in a working day
(high productivity) using Li-Cor and
Lumex instruments. Note that the Li-Cor
gas detector is specifically designed to
assess advective fluxes. It makes it
possible to assess the importance of gas
leaks in sectors deemed to be anomalous in
He and H2.
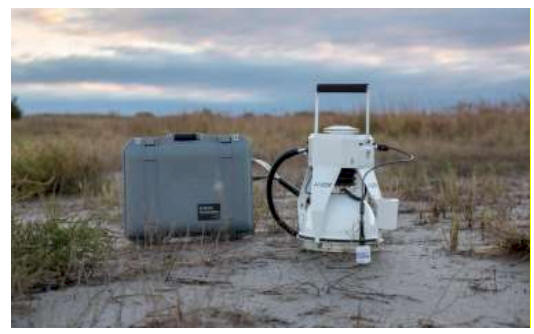
Figure 12.
Portable 7810 Li-Cor detector coupled to a
gas flow chamber.
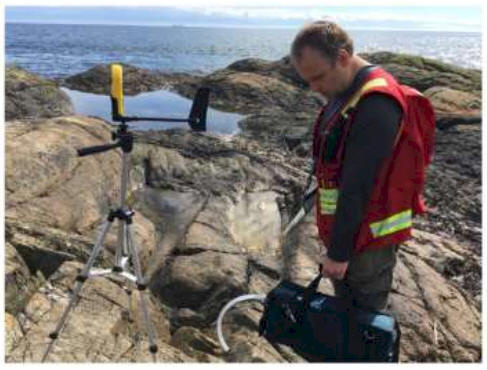
Figure 13. Lumex
gaseous mercury detector. Field measurements
in British Columbia (Rukhlov et al., 2021).
4.1.2.2 Soil-Gas
geochemistry in the soil (vadose zone)
Soil-Gas geochemistry
is based on the sampling of gas present in
the water[1]unsaturated
zone of the soil (vadose zone). Using a
probe specially designed for gas sampling in
soils, gas samples are taken at a depth of
about 1m in the ground. Such gas sampling is
possible in relatively dry weather
conditions (summer). Since H2 is
more concentrated in nature than helium, its
detection can be done more quickly in the
field using portable gas detectors (e.g.,
H2 specific electrochemical
detector). Since helium cannot be easily
measured in real time, its precise analysis
(with less than 1 ppm precision) must be
deferred over time and requires
spectrometric analysis in the laboratory.
However, helium analyses, with a limit of 2
ppm, can be carried out in the field using
the Agilent PHD 4 technology. PHD 4
analyzes are useful for locating advective
leaks and anomalous regional areas but for
diluted diffusive gas concentrations (near
background level), the detection limit maybe
too high. However, conclusive regional
studies have been successfully carried out
with this method, which could be
advantageously used in the Témiscamingue
area, especially in the second phase of the
soil-gas surveys and more specifically to
densify the data in the vicinity of
anomalous sectors in He detected during the
first phase of soil-gas survey.
In the field, other
gases such as H2S, SO2,
CS2, O2, and
possibly radon should be analyzed in order
to optimize the process of detecting sites
favourable to the presence of helium and
hydrogen (Fig. 14). The analysis of
these gases also makes it possible to
specify the characteristics of the source
regions from which the gases come. Since
these gases are measured with the same
detector as for hydrogen, the analysis of
H2S, SO2, CS2,
O2, does not increase the
analytical costs.
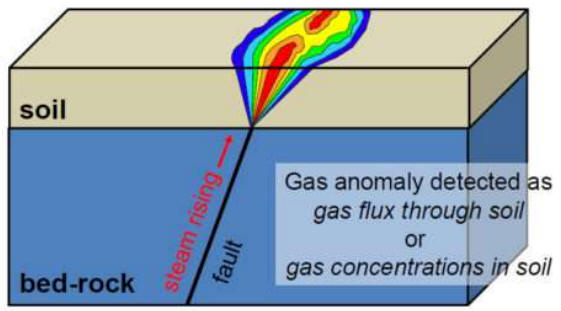
Figure 14. Gas
anomaly related to the presence of a fault.
Soil-Gas sampling
procedures is a relatively well-mastered
technique in environment, soil sciences and
natural resources exploration for the search
for hydrocarbons, helium, hydrogen and
sulphide mineralisations (Fig. 15 and 16).
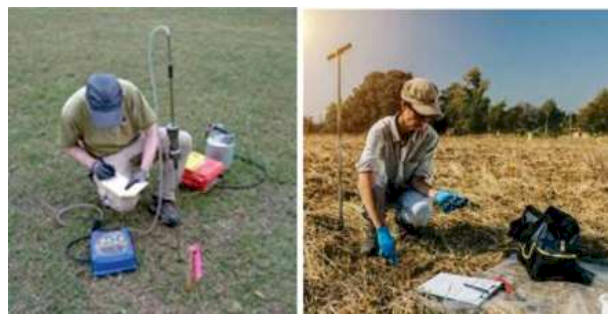
Figure 15.
Photographs showing different gas sampling
procedures in soils.
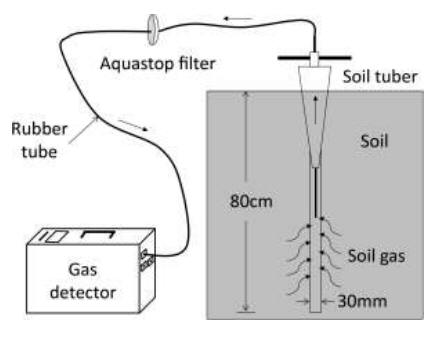
Figure 16.
Real-time gas sampling device in the vadose
zone of a soil (Soil-Gas method).
To ensure quality
control over the soil gas sampling process,
it is important to accurately monitor the
local meteorology, paying particular
attention to monitoring precipitation and
soil moisture. Thus, an autonomous weather
station should be installed within the
perimeter of the Soil-Gas surveys in the
Témiscamingue area.
4.2. FIELD GEOPHYSICS
As for natural gas
exploration, it is imperative to carry out
geophysical surveys in order to validate the
interpretations of gas geochemical surveys.
Geophysical data are very important as they
enable faults and lithological contacts to
be located with precision. In addition,
these data and inversion models make it
possible to verify the vertical penetration
of the faults and to visualize the presence
of anticlinal folded structures, which are
favourable to the storage of gases.
4.2.1 Compilation of
geological and geophysical data
For QIMC exploration
projects in Témiscamingue, a compilation of
the geological and geophysical data
available on the SIGEOM site of the MRNQ
should be carried out. This data should be
compiled on GIS so that it can be easily
used for planning fieldwork and interpreting
gas geochemistry.
4.2.2 Ground-based
gamma radiometric surveys
In the subsurface,
areas of high gas concentrations are often
associated with oxygen-deficient
environments and therefore with reducing
redox potential of soils. These conditions
allow the precipitation of natural uranium
because this element is mobile in the
context of oxygenated water and precipitates
in a reducing medium. Over time, groundwater
passing near the gas vent will precipitate
uranium creating an anomaly characterized by
uranium enrichment. Uranium enrichments near
faults have often been reported in the
scientific literature and during soil-gas
surveys for natural gas exploration. These
halos can be detected by ground-based mobile
gamma spectrometers using ATVs or trucks.
The latter are coupled with GPS in order to
be able to map the terrain in real time (IAEA,
2003). For example, the gamma detectors
used by INRS (RS[1]700
system) for ground surveys are similar to
those used in helicopter-borne surveys
(Radiation Solutions). Thus in real time,
soils rich in U, K or Th can be detected and
interpreted for prospecting hydrogen and
helium and also to locate the different
geological units such as between limestone,
quartzite and arkose which are present in
the Temiscamingue area.
For the radiometric
surveys, we suggest using two gamma
detectors coupled to a spectrometer and a
Trimble DGPS allowing acquisition in
continuous mode (one K-eU-eTh determination
per second). Devices such as the RS-700
system of Radiation Solutions Inc.
(Mississauga, Ontario) is very efficient for
such purpose (Fig. 17).
The INRS RS-700
system comprises the following
components:
- Two 4
litres-256 cubic inches RSX-1 gamma
detectors (NaI
- sodium iodide
crystal)
- Trimble
integrated GPS system
- RS 701
spectrometer with a spectral resolution of
1024 channels
- Panasonic
Toughbook laptop
- Mule Kawaski
Diesel ATV

Figure 17.
RS-700 mobile gamma spectrometric system
(Radiation Solutions inc).
4.2.3
Audiomagnetotelluric survey (AMT)
The search for faults
or geological contexts favourable to the
presence of gas (e.g., reservoir rocks with
impermeable sedimentary or volcanic cover)
requires the use of geophysical imaging
technologies with high vertical penetration.
Frequency-based electromagnetic methods
using natural sources such as the AMT method
and seismic methods are particularly
effective for probing the ground in depth.
For the Temiscamingue project, we suggest
using the AMT method because of its low cost
of use, the safety during field operations
(no explosives, no generators with strong
electric currents on the ground). This
method was successfully used for Falco
Resources in the western part of the Noranda
mining camp (Richer-LaFlèche et Pilote,
2018; FRQNT-MRN-Mines research grant). The
INRS team successfully detected new faults
along an 8,5 km long section of the Archean
Blake River Group (Fig. 18). This
demonstrates the performance of the AMT
detection and imaging method.

Figure 18. Example of the AMT survey
(INRS) of the Lac Flavrian road (Rouyn-Noranda)
carried out under the FRQNT-MERN-Mines grant
in collaboration with Falco Mining
Resources. Note the location of the reverse
fault (gold sector). Source: Richer-LaFlèche
and Pilote (2018).
4.2.4 Gravity survey
The gravimetric method is very useful in gas
exploration in order to delimit the presence
of thick accumulations of sedimentary rocks.
At the regional scale, the gravity troughs
correspond to sedimentary accumulations (low
density rocks) and the gravity highs often
correspond to the rise of the Precambrian
basement (dense rocks). For the search of H2
and He reservoirs in Témiscamingue, this
method could be very useful for locating the
maximum thicknesses of the sedimentary rocks
of the Cobalt Group and those of the
Ordovician rocks. Additionally, gravity data
can be inverted in 2D and 3D to produce
realistic geological models. Furthermore,
gravimetry is highly sensitive to faults
juxtaposing rocks of different natures. In
addition, the worming modeling method, which
calculates the position of faults and their
geometry (dip and depth), is very effective
when the spatial density of gravimetric
measurements is sufficient in a region.
Figure 19 shows the relationship between
gravity troughs and natural gas accumulation
in soils resting on the Devonian rocks of
Témiscouata Formation (Appalachians of
Quebec).
For gravimetric measurements, a CG5
gravimeter (Scintrex, Ontario) could be used
to measure ground data. An RTK GPS system
will then be used to determine the position
and especially the elevation of the gravity
stations with a vertical precision of less
than 5 cm.

Figure 19. Gravity trough, thickening
of a sedimentary basin and gas accumulation
in the St-Eusèbe sector (Témiscouata):
Source: Richer-LaFlèche (2008; 2011). Note
that ethane is used, instead of methane,
because it is only produce from thermogenic
process.
4.3 LIDAR IMAGERY AND
HIGH SPATIAL RESOLUTION TOPOGRAPHY
For the search for hydrogen and helium, as
for natural gas, the detection of faults or
other structural discontinuities (e.g.,
joint, diaclase) is important in order to
locate potential sites of advection of gas
(seepage). In a tectonically active region,
such as the Timiskaming rift and graben
system, recently reactivated faults can emit
appreciable amounts of gas. The location of
these faults is complicated by the presence
of quaternary deposits (tills), of forest,
agricultural and forest soils masking the
bedrock and therefore the faults
intersecting the rock. In such a context,
drone Lidar surveys provide precise data of
the surface elevation of soils and rocky
outcrops with an accuracy of the order of a
few centimeters and a point density of the
order of 50 to 100 points of measure per m 2
. The Lidar imagery, presented in Figure
20, shows examples of Lidar data (low
resolution) for the Pontiac (Kipawa and
Angliers areas) and Charlevoix sectors (Malbaie
area). The St-Bruno-de Guigues sector could
be the subject of a high spatial resolution
Lidar drone survey. However, given the
presence of a forest that is often rich in
deciduous trees, it would be preferable to
carry out the survey after the snow melts
and before the leaves come out (year 2
exploration program).
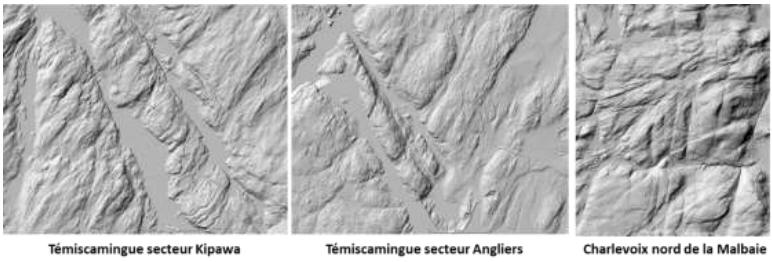
Figure 20. Lidar imagery of the
Kipawa and Angliers sectors (Pontiac) and
northern Malbaie (Charlevoix): Source: Forêt
Québec.

Figure 21. Fault detection by SAR
satellite imagery and drone Lidar.
5. DESCRIPTION OF WORK
TO BE PERFORMED FOR H2 AND He
EXPLORATION IN THE ST-BRUNO-DE-GUIGUES AREA
(TÉMISCAMINGUE PROJECT)
The exploration work schedule, suggested for
the Témiscamingue property, is presented
briefly in the form of a Gantt chart on
figure 22.
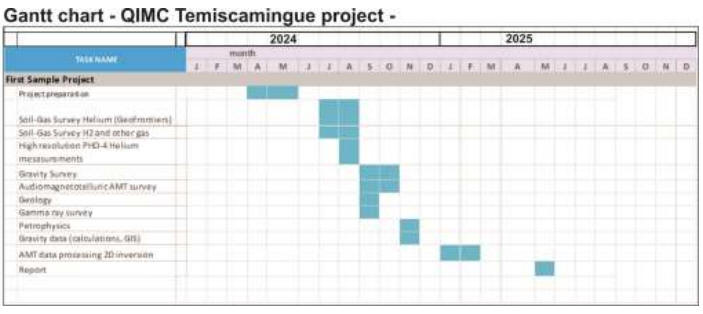
Figure 22. Suggested planning for
exploration work for hydrogen and helium on
QIMC's Témiscamingue property.
5.1 GRAVITY SURVEY (survey time: 20 days or
771 stations)
The CG5 gravimetric survey (Fig. 23)
should be done along the main secondary
roads and access trails of the study area (Fig.
24). The spacing of the stations, along
the roads and trails, should be around 100
m. The stations should be surveyed with a
GPS RTK system with accuracy at the
centimetre level. About 771 gravity stations
could be measured in the St-Bruno-de-Guigues
area. The gravimetric station of the
Notre-Dame-du-Nord church should be used in
order to establish a new reference station (QIMC
station) in the municipality of St-Bruno[1]de-Guigues.
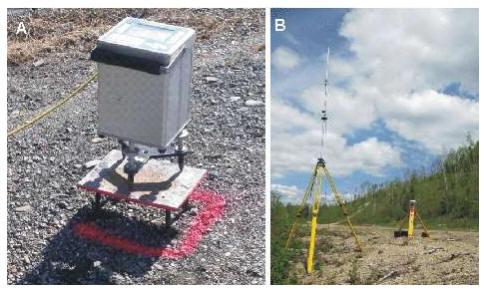
Figure 23. a) Scintex CG-5 gravimeter
and b) RTK base station with Pacific Crest
radio.

Figure 24. Proposed gravimetric
survey lines in the St-Bruno-de Guigues area
(QIMC’s Témiscamingue project).
5.2 SOIL-GAS SURVEYS
Soil-gas sampling (Fig. 25) will be
carried out along many sections of the
Témiscamingue property (Fig. 26). For
this project, two sampling phases will be
necessary. The first (phase 1)
consists of a regional survey requiring very
high precision measures (mass spectrometry)
in order to be able to detect domains with
diffusive anomalies of helium. The soil-gas
samples will have to be shipped to the
Geofrontiers laboratory (Texas) for the
measurements of helium with analytical
precision under the ppm level. Subsequently,
when the anomaly sectors will be detected,
high spatial resolution sampling (with
spacing of 25 m) will be carried out with
the PHD-4 portable helium detector (in situ
measurements) (phase 2). Although the
PHD-4 detector has a detection limit of 2
ppm, it was frequently used to specify the
location of economically significant seepage
of helium.
5.2.1 Soil-gas surveys
An
average inter-sample distance of 100 m will
be used as a linear sampling for the phase 1
surveys. In some cases, the spacing will be
greater due to the presence of obstacles
preventing sampling (e.g., watercourses,
flood zone, residential sector). In this
project, we have chosen the method of
analyzing the free gas fraction present in
the vadose zone (not saturated with water)
of the soil to assess the helium potential
of the Témiscamingue property.
Phase 1 high precision helium sampling
(N= 250 samples): The gas samples for
helium will be taken using steel probes from
Geofrontiers (USA) specifically designed for
the sampling of soil-gas. The probe will be
implemented about 1m in the ground using a
vertical axis striker fixed at the top of
the probe. Following the insertion of the
probe at the desired depth, a syringe will
be inserted into an airtight septum located
in the upper part of the probe. A first
volume of gas equivalent to that of the
internal tube of the probe will be taken and
then eliminated in order to purge the probe
from all traces of previous samples or
atmospheric air accumulated during the
transport of the probe. Once this step is
completed, the operator will take a second
sample of 20 cc and insert the latter in a
gas chromatography bottle for analysis (Fig.
26). Laboratory trials have shown that
gas bottles retained the prescribed vacuum
and helium for a period of more than a
month.
Phase 2 normal Helium survey (N= 250
samples): Following the obtaining of the
analytical results from Geofrontiers (USA)
and the implementation of the data on GIS,
we will target the anomalous sectors to be
sampled in high resolution mode. For this
task, we will use the PHD-4 detector which
can directly measure Helium concentrations
in the field. 25 m measurements and if
necessary 10 m measurements will be taken in
order to locate the helium leaks responsible
for the formation of the diffuse anomalies
detected in phase 1 of the helium survey.
Sampling of hydrogen and other gases (N=
517 samples): Sampling specific to
hydrogen and to other gases (CO2, SO2, H2S,
N2, etc.), with measurements directly in the
field, will be carried out during the first
year of the exploration program. The
sampling method resembles that for helium
(soil-gas). However, the sampling procedure
(pre-drilling, use of a conical auger) and
the in situ gas analysis are significantly
different. The number and position of field
measurement stations will be similar to the
helium survey.
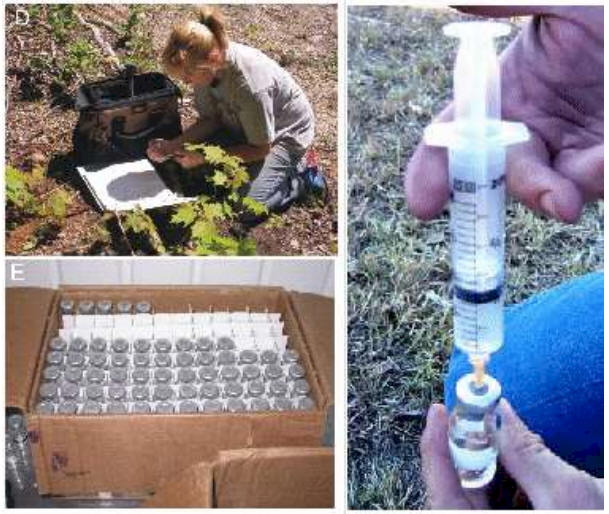
Figure 25. Photographs showing
different stages of the helium sampling
process using the soil-gas method.
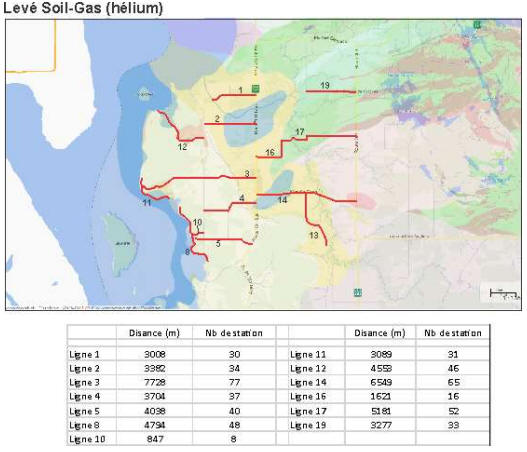
Figure 26. Soil-gas survey for helium
(red lines) in the Témiscamingue QIMC
property area (St-Bruno-de-Guigues).
5.3. GAMMA RADIOMETRIC SURVEY (7 DAYS
SURVEY)
Mobile ground-based gamma spectrometry
should be used because of its ability to
cover, with a high density of points,
significant linear distance. K%, eU ppm and
eTh ppm concentrations could be acquired at
a rate of one series of measurements per
second. This data could be coupled in real
time to a Trimble GPS. The acquisition could
be carried out using a Kawasaki Diesel mule
or other type of ATV. As mentioned in the
text, the data could be used to 1) locate
reducing halos associated with the presence
of gas leaks in the soil and 2) improve
local geological mapping by identifying
units rich (e.g., shales) or poor in K and
Th (e.g., limestone). As part of this
project, the gamma radiometric system could
be used for a period of 7 days.
5.4
AUDIOMAGNETOTELLURIC SURVEY (8 DAYS SURVEY)
The audiomagnetotellurics (AMT) survey is
essential to: 1) understand the deep geology
of the Temiscamingue graben, 2) to locate
the regional faults, 3) to evaluate the
importance of the faults (e.g., vertical
penetration in the crust) and 4) to study
the relationship between the position of the
faults and that of the soil[1]gas
anomalies. The AMT survey should be carried
out in scalar mode and in continuous
profiling with measuring stations at a 50 m
spacing. Such high resolution way of
measuring AMT data makes it possible to
produce continuous geoelectrical imagery.
The latter is essential in order to locate
faults which are generally very narrow
structures.
A
Zonge GDP32-24bits receiver can be used with
an ANT/4 magnetic antenna (Fig. 27)
to do the AMT measurements in the field. For
carrying out the AMT survey we suggest the
following procedure:
The AMT setup will include two lines of 200
m (with 8 Ex), arranged on either side of
the GDP32/II receiver. Four groups of
electrodes distributed at regular intervals
of 50 m will be linked together by each
telluric line. The measurements will be
performed in series using 8 groups of
electrodes installed at a time and the
magnetic receiver (Hy) will be located in
the middle of the acquisition line (Fig.
28). The magnetic receiver will be
oriented perpendicular to the survey line.
Each sequence of measurements, or sampling
stations, will thus be spread over 400 m in
length. The positions of each electrode (and
the receiver of each measuring station) will
be located using a GPS device. Non-polarizable
ceramic electrodes (with copper sulphates)
will be used to accurately measure the
electric field (Ex). Remember that the AMT
method is based on the measurement of
impedance (Z), i.e. the electric
field/magnetic field ratio (or Z = E/H).
Electric field values are obtained by
measuring the potential difference between
the ends of electrodes driven into the
ground and dividing this value by the length
of the dipole. The magnetic field is
measured using a magnetic receiver, or
antenna, made of a coil of mu-metal wire.

Figure 27. Zonge audiomagnetotelluric
system with GDP32-24 bits receiver, porous
ceramic electrodes and ANT/4 magnetic
sensors.

Figure 28. Scalar
audiomagnetotellurics (AMT) setup with 8 Ex
stations at 50 m spacing that will be used
for surveys in the Témiscamingue area.
5.5 LIDAR AND PHOTOGRAMMETRIC DRONE
SURVEY (5 DAYS SURVEY)
This survey will be very useful to document
the presence of faults or sub-circular
depressions on the ground. However, the
Lidar survey should be done in late fall
(after leaf fall and before snowfall) or in
early spring (after snowmelt). Given the
immensity of the St-Bruno-de-Guigues
property and Transport Canada's flight
limitations of 500m (visual flight), the
surveys will be carried out by carrying out
measurements in corridors centred on the
soil-gas survey lines. The measurements will
therefore be carried out 250m on either side
of the soil-gas survey lines. The airport
area will not be able to be measured due to
Transport Canada's drone flight bans. A
Viewprotech M115 drone and an AlphaAir 450
Lidar will be used for the Lidar surveys.
5.6 MOBILE LASER
DETECTOR GAS SURVEY (10 DAYS)
In
the framework of this project, we will use a
mobile platform coupled with a precision GPS
in order to be able to detect areas of
degassing of the rock and soil with gas
measurements of the order of a second. This
technique will use gases such as CO2
and/or CH4 because these
molecules are very sensitive to detectors
based on the use of lasers.
The objective of these surveys is to
measure, using an ATV, a large number of
forest or agricultural roads and trails in
order to detect advective (higher flow) gas
leaks. These leaks, which are often less
than 10m in size (at the surface), are often
associated with porous geological faults
that promote the circulation of high-flow
gases. Once detected, these areas will be
prioritized and will be subject to a large
number of Soil-Gas measurements (vadose
zone) in order to check for the presence of
H2 and He. The second objective of these
mobile surveys is not to miss an advective
area during the normal soil-gas survey
because sampling interval is in the range of
100m during a regional survey.
A
high-performance technology to relay this
task is that of the Canadian company Boreal
Laser. The latter is shown in figure 29.

Figure 29. CO2 or CH4
detection system based on the use of a laser
source. Images taken from the Boreal
website.
6. SUMMARY OF COSTS
RELATED TO EXPLORATION WORK ON THE
TEMISCAMINGUE PROJECT
-Mobilization-demobilization
(summer and fall): $15 299
-Audiomagnetotelluric survey: $
55 602
-Gravity survey and RTK
surveying: $ 82 776
-Petrophysical measurements: $ 5
264
-Geology: $ 13 265
-Soil-gas survey for helium
(phase 1): $ 65 263
-Soil-gas survey for helium
(phase 2): $ 20 250
-Soil-gas survey for H2 and
other gases: $ 63 901
-Gamma radiometric survey: $ 23
177
-Weather stations: $ 5 600
-Mobile laser gas survey (+GPS):
$ 24 710
-Report on soil-gas
geochemistry: $ 15 000
-Lidar (drone) and aerophoto: $
25 075
Total (with administrative costs): $ 415 182 |
|